Continuous Wave Radar
Principle of Operation
As opposed to pulsed radar systems, continuous wave (CW) radar systems emit electromagnetic radiation at all times. Conventional CW radar cannot measure range because there is no basis for the measurement of the time delay. Recall that the basic radar system created pulses and used the time interval between transmission and reception to determine the target's range. If the energy is transmitted continuously then this will not be possible.CW radar can measure the instantaneous rate-of-change in the target's range. This is accomplished by a direct measurement of the Doppler shift of the returned signal. The Doppler shift is a change in the frequency of the electromagnetic wave caused by motion of the transmitter, target or both. For example, if the transmitter is moving, the wavelength is reduced by a fraction proportional to the speed it is moving in the direction of propagation. Since the speed of propagation is a constant, the frequency must increase as the wavelength shortens. The net result is an upwards shift in the transmitted frequency, called the Doppler shift.
Figure 1. Doppler shift from moving transmitter
Likewise, if the receiver is moving opposite to the direction of propagation, there will a increase in the received frequency. Furthermore, a radar target which is moving will act as both a receiver and transmitter, with a resulting Doppler shift for each. The two effects caused by the motion of the transmitter/receiver and target can be combined into a net shift the frequency. The amount of shift will depend of the combined speed of the transmitter/receiver and the target along the line between them, called the line-of-sight (LOS).
Figure 2. Calculating the relative speed in the line-of-sight.
The Doppler shift can be calculated with knowledge of the transmitter/receiver and target speeds, here designated as s1 and s2 respectively, and the angles between their direction of motion and the line-if-sight, designated q1 and q2. The combined speed in the line-of-sight is
s = s1 cosq1 + s2 cosq2 .
This speed can also be interpreted as the instantaneous rate of change in the range, or range rate. As long as the problem is confined to two-dimensions, the angles also have simple interpretations: q1 = the relative bearing to the target. The difference between the course of the transmitter/receiver and the true bearing to the target. This follows the old nautical rule:
Relative Bearing = True Bearing - Heading
Due to the characteristics of the cosine function, it makes no difference whether angle is positive or negative (strictly speaking, relative bearings are always positive and range from 0 to 3590). q2 = the target angle (relative bearing of transmitter/receiver from target). Computed in an identical manner as the relative bearing, except that the target's course is substituted for the heading and the reciprocal bearing is used instead of the true bearing to the target. The reciprocal bearing is found by:
Reciprocal Bearing = True Bearing 1800
Again, it does not matter is this result is positive, negative or even beyond 3600, although the proper result would be in the range of 0-3590. Assuming that the range rate is known the shift in returned frequency is
Df = 2s/l
where l is the wavelength of the original signal. As an example, the Doppler shift in an X-band (10 GHz) CW radar will be about 30 Hz for every 1 mph combined speed in the line-of-sight.
Example: speed gun.

Police often use CW radar to measure the speed of cars. What is actually measured is the fraction of the total speed which is towards the radar. If there is some difference between the direction of motion and the line-of-sight, there will be error. Fortunately for speeders, the measured speed is always lower than the actual.
CW radar systems are used in military applications where the measuring the range rate is desired. Of course, range rate can be determined from the basic pulsed radar system by measuring the change in the detected range from pulse to pulse. CW systems measure the instantaneous range rate, and maintain continuous contact with the target.
Frequency Modulated Continuous Wave (FMCW) Radar
It is also possible to use a CW radar system to measure range instead of range rate by frequency modulation, the systematic variation of the transmitted frequency. What this does in effect is to put a unique "time stamp" on the transmitted wave at every instant. By measuring the frequency of the return signal, the time delay betweentransmission and reception can be measure and therefore the range determined as before. Of course, the amount of frequency modulation must be significantly greater than the expected Doppler shift or the results will be affected.
The simplest way to modulate the wave is to linearly increase the frequency. In other words, the transmitted frequency will change at a constant rate.
Figure 3. FMCW theory of operation.
The FMCW system measures the instantaneous difference between the transmitted and received frequencies, Df. This difference is directly proportional to the time delay, Dt, which is takes the radar signal to reach the target and return. From this the range can be found using the usual formula, R = cDt/2. The time delay can be found as follows:
Dt = T Df/(f2-f1) where:
f2 = maximum frequency
f1 = minimum frequency
T = period of sweep from f1 to f2,
and Df = the difference between transmitted and received.
There is a slight problem which occurs when the sweep resets the frequency and the frequency difference becomes negative (as shown in the plot of Df vs. time). The system uses a discriminator to clip off the negative signal, leaving only the positive part, which is directly proportional to the range. 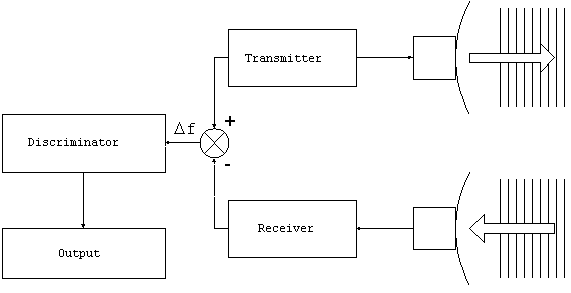
Figure 4. FMCW block diagram.
Combining these equations into a single form for the range R = 2cTDf/(f2 - f1)
where Df is the difference between the transmitted and received frequency (when both are from the same sweep, i.e. when it is positive).
Another way to construct a FMCW system, is to compare the phase difference between the transmitted and received signals after they have been demodulated to receiver the sweep information. This system does not have to discriminate the negative values of Df. In either case however, the maximum unambiguous range will still be determined by the period, namely
Runamb = cT/2
FMCW systems are often used for radar altimeters, or in radar proximity fuzes for warheads. These systems do not have a minimum range like a pulsed system. However, they are not suitable for long range detection, because the continuous power level they transmit at must be considerably lower than the peak power of a pulsed system. You may recall that the peak and average power in a pulse system were related by the duty cycle,
Pave = DC *Ppeak
For a continuous wave system, the duty cycle is one, or alternatively, the peak power is the same as the average power. In pulsed systems the peak power is many times greater than the average.
http://www.fas.org/man/dod-101/navy/docs/es310/cwradar/cwradar.htm
Christian Argenis Umaña Zambrano
ci :17678077